Quantum Sensors: Unlocking the Future of Measurement
Quantum sensors represent a paradigm shift in measurement capabilities, pushing the boundaries of what is possible. Their ability to harness the peculiarities of quantum mechanics opens up a world of opportunities for high-precision measurements and transformative applications.
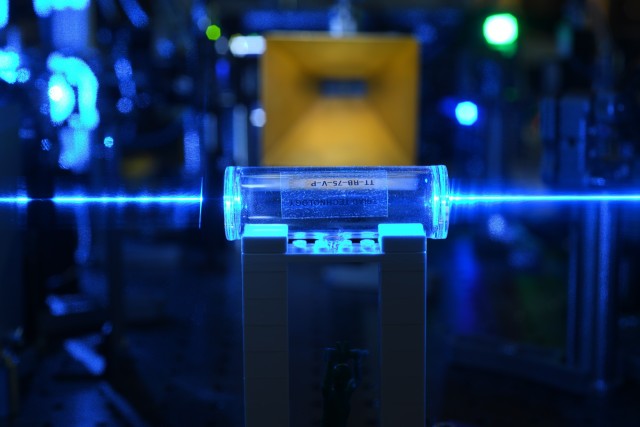
Quantum sensors are a groundbreaking technology that holds immense potential for revolutionizing various fields, including metrology, navigation, healthcare, and environmental monitoring. By harnessing the principles of quantum mechanics, these sensors offer unparalleled sensitivity and precision, surpassing the capabilities of classical sensors.
The Basics of Quantum Sensors
Quantum sensors operate on the fundamental principles of quantum mechanics, a branch of physics that describes the behavior of particles at the atomic and subatomic levels. These sensors utilize the unique properties of quantum mechanics to achieve remarkable levels of sensitivity and precision in measuring various physical quantities.
At the heart of quantum sensors are two key principles: quantum superposition and entanglement.
Quantum Superposition: According to quantum mechanics, particles can exist in multiple states simultaneously. This phenomenon is known as superposition. In the context of quantum sensors, superposition allows particles to be in different states at the same time, which enables more accurate measurements.
Entanglement: Entanglement is another intriguing property of quantum mechanics. When particles become entangled, their states become correlated, regardless of the physical distance between them. Changes in one particle's state instantaneously affect the state of the other, even if they are light-years apart. This phenomenon opens up new possibilities for measurement and communication.
Quantum Superposition and Entanglement
Quantum superposition allows particles to exist in multiple states at once, which can be exploited in quantum sensors to enhance measurement precision. By preparing particles in a superposition state, sensors can effectively increase the probability of detecting a specific measurement outcome, resulting in superior sensitivity.
Entanglement, on the other hand, enables the correlation of quantum states between particles. This property allows quantum sensors to detect minute changes in physical quantities by measuring the resulting alterations in the entangled particles, even when they are physically separated.
Quantum Sensing Mechanisms
Quantum sensors employ various mechanisms to measure physical quantities with exceptional precision. These mechanisms leverage the principles of quantum mechanics to extract valuable information from the quantum world. Let's explore some of the key quantum sensing mechanisms:
Interferometry: Interferometry is a widely used technique in quantum sensing. It exploits the wave-like nature of particles, such as photons or atoms, to measure changes in phase or frequency. By splitting a quantum particle into two paths and then recombining them, interference patterns are created. These patterns contain valuable information about the measured quantity, allowing for highly accurate measurements.
Interaction with Quantum Systems: Quantum sensors also rely on the interaction between quantum systems and external fields. For example, a quantum sensor may utilize the interaction between atoms or molecules and magnetic fields, electric fields, or gravitational fields. By measuring the effects of these interactions, the sensor can deduce the desired physical quantities.
Quantum Spin: Quantum spin is an intrinsic property of particles, such as electrons or atoms, which gives rise to magnetic moments. Quantum sensors can measure changes in the orientation or alignment of these spins to detect magnetic fields or other related quantities. This mechanism is particularly useful in applications such as magnetic field mapping and magnetic resonance imaging (MRI).
Quantum Metrology: Quantum metrology involves using entangled quantum states to achieve enhanced measurement precision. Entangled states allow for more accurate estimation of physical quantities, surpassing the limits imposed by classical measurements. Quantum metrology has the potential to revolutionize fields such as timekeeping, distance measurement, and gravitational wave detection.
Types of Quantum Sensors
Atom Interferometers
Atom interferometers employ the wave-like behavior of atoms to measure parameters such as acceleration, rotation, and gravitational forces. By splitting a cloud of ultra-cold atoms and recombining them after subjecting each path to different conditions, interference patterns can be observed and analyzed to determine the desired physical quantities.
Nitrogen-Vacancy Centers
Nitrogen-vacancy (NV) centers are defects found in diamond crystals that possess remarkable sensing capabilities. NV centers can detect and measure temperature, magnetic fields, and electric fields with exceptional precision. This property makes them promising candidates for applications in quantum computing, biomagnetism, and nanoscale imaging.
Superconducting Quantum Interference Devices (SQUIDs)
Superconducting Quantum Interference Devices (SQUIDs) are extremely sensitive magnetometers that utilize superconducting loops to measure minute changes in magnetic fields. SQUIDs find applications in diverse fields, including geophysics, materials characterization, and neuroscience, where high sensitivity is crucial.
Optical Gravitational Wave Detectors (OGWDs)
Optical Gravitational Wave Detectors (OGWDs) are sensors designed to detect gravitational waves by utilizing the interference of laser beams. These detectors can offer unprecedented sensitivity and are essential for studying astrophysical phenomena and advancing our understanding of the universe.
Applications of Quantum Sensors
Quantum sensors have a wide range of applications across various domains. Some notable applications include:
Precision Measurements
Quantum sensors enable ultra-precise measurements of physical quantities such as time, distance, gravity, and magnetic fields. These capabilities have significant implications in fields such as geodesy, geophysics, and fundamental physics research.
Quantum Navigation and Inertial Sensing
Quantum sensors can revolutionize navigation systems by providing precise position, orientation, and acceleration information. Applications include autonomous vehicles, robotics, and aerospace.
Medical Diagnostics and Imaging
Quantum sensors have the potential to enhance medical diagnostics by enabling high-resolution imaging techniques, early disease detection, and monitoring physiological processes at the molecular level.
Environmental Monitoring
Quantum sensors offer the ability to monitor and analyze environmental parameters with extraordinary precision. This includes monitoring air quality, detecting pollutants, and studying climate change indicators.
Advantages and Challenges of Quantum Sensors
Quantum sensors offer a range of advantages over classical sensors, but they also face unique challenges. Let's explore the advantages and challenges associated with quantum sensors:
Advantages:
Unprecedented Sensitivity: Quantum sensors provide unparalleled sensitivity, enabling the detection of extremely small changes in physical quantities. This sensitivity allows for precise measurements that were previously unattainable with classical sensors.
High Precision: Quantum sensors leverage the principles of quantum mechanics to achieve exceptional precision. They can measure physical quantities with remarkable accuracy, enabling scientists and engineers to delve into previously unexplored realms of measurement.
Enhanced Selectivity: Quantum sensors can exhibit high selectivity, meaning they can differentiate between closely related quantities or identify specific characteristics of a system. This selective capability opens up possibilities for advanced analysis and diagnostics.
Broad Measurement Range: Quantum sensors can cover a wide range of measurement scales, from the nanoscale to astronomical distances. This versatility makes them valuable in diverse fields, including fundamental research, medical diagnostics, and environmental monitoring.
Challenges:
Technical Complexity: Quantum sensors often require sophisticated experimental setups and intricate control systems. The manipulation and measurement of quantum states demand specialized knowledge and advanced technological infrastructure, which can pose challenges in practical implementation.
Cost: Quantum sensors can be costly to develop and manufacture due to the specialized components and precise engineering involved. The high cost of materials, experimental equipment, and skilled personnel can hinder their widespread adoption and commercial availability.
Environmental Stability: Quantum sensors are highly sensitive to environmental factors such as temperature, electromagnetic fields, and vibrations. Maintaining stable and controlled conditions is essential for accurate measurements. Ensuring environmental stability can be challenging, particularly in real-world applications outside controlled laboratory settings.
Limited Integration: Integrating quantum sensors into existing systems and devices can be challenging. The compatibility and scalability of quantum technologies with other components and platforms need to be addressed for seamless integration and practical utilization.
Future Outlook: Quantum Sensor Technologies
The future of quantum sensors looks promising, with ongoing research and development efforts aimed at improving their performance, reducing costs, and expanding their applications. Advancements in quantum computing, nanotechnology, and materials science are expected to drive the evolution of quantum sensor technologies.
Quantum sensors represent a paradigm shift in measurement capabilities, pushing the boundaries of what is possible. Their ability to harness the peculiarities of quantum mechanics opens up a world of opportunities for high-precision measurements and transformative applications. As research and development continue to progress, quantum sensors are poised to revolutionize multiple industries, paving the way for a future where measurement precision knows no bounds.
FAQs
Q: Are quantum sensors only useful in scientific research?
A: No, quantum sensors have diverse applications in various fields, including healthcare, navigation, and environmental monitoring.
Q: Can quantum sensors improve the accuracy of GPS?
A: Yes, quantum sensors have the potential to enhance the accuracy and reliability of GPS systems, enabling more precise positioning information.
Q: Are quantum sensors commercially available?
A: While some quantum sensors have made their way to the market, widespread commercial availability is still in its early stages due to ongoing research and development.
Q: Can quantum sensors be miniaturized?
A: Yes, efforts are underway to develop miniaturized quantum sensors that can be integrated into compact devices and systems.
Q: Will quantum sensors replace classical sensors entirely?
A: Quantum sensors offer unique capabilities, but they are not expected to replace classical sensors entirely. Both types of sensors will coexist, with each being suited for specific applications.
What's Your Reaction?
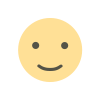
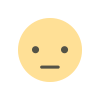
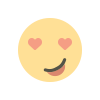
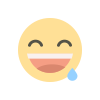
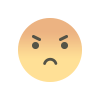
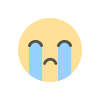
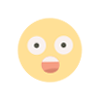